Introduction
Lung cancer is one of the most prevalent cancers worldwide, with the highest cancer-related mortality rate. Confirmation of the diagnosis of both pulmonary and pleural lesions by histopathological examination is essential to facilitate the implementation of an appropriate therapeutic strategy. However, the viability of bronchoscopic biopsy is limited when lesions are not confined to the airways or larger bronchi. In contrast, computed tomo-graphy (CT)-guided biopsy is emerging as the dominant diagnostic modality and is widely used to differen-tiate between benign and malignant lung and pleural le-sions [1,2]. The characteristics of lung tissue, which contains large amounts of air and a dense network of blood vessels, make it particularly susceptible to complications during the procedure. One of the most common complications of CT-guided lung biopsy is the development of pneumothorax, occurring in 8 to 69% of cases [3]. However, due to the self-limiting nature of pneumothorax, most patients who are diagnosed with this condition do not require any further intervention. Another typical complication of the procedure is pulmonary haemorrhage, which is observed in approximately 18% of cases [4]. Other adverse events related to CT-guided biopsy may include haemoptysis, needle tract seeding, systemic air embolism, haemothorax, and death [5,6].
Given the requirement for multiple scans to target the lesion, the planning sequence, and a control examination to ensure there are no complications, the dose for CT-guided biopsy is quite high (up to 40 times higher than regular diagnostic chest CT) [7]. Based on experience with certain low-dose CT protocols used in many other clinical procedures, dose reduction strategies for CT-guided biopsies have been investigated to reduce radiation exposure to patients and medical staff [8]. The dia-gnostic accuracy of CT-guided lung biopsy is estimated to be 64–97%. At present, low-dose, CT-guided lung biopsies are used in daily clinical practice, particularly as the method of choice for peripherally located lesions of 2 cm or less in diameter.
Initially, an ultra-low-dose protocol for CT-guided lung biopsy was 120 kV tube voltage (120 kV localization/120 kV subsequent guide scans) with auto-mA (usually 30-100 mAs) [9]. However, recently the ultra-low-dose CT-guided lung biopsy protocols have changed to 100 kV or 80 kV and an electric intensity of 7.5 mAs or even less. As a result, there is a significant reduction in the mean radiation dose for biopsy [10]. The median radiation dose ranges from 10.98 mGy cm to 133.3 mGy cm [8].
The aim of this paper is to evaluate the efficacy and safety of a low-dose, CT-guided transthoracic biopsy of lung and pleural lesions.
Material and methods
A total of 135 consecutive low-dose, CT-guided transthoracic biopsies of lung and pleural lesions were performed in 135 patients between 2018 and 2021. The analysis included 55 females and 80 males, aged 32 to 91 years, with a mean age of 66 years. Patients were enrolled for the biopsy after prior thoracic-surgical and radiological consultations. Informed consent was obtained from all subjects eligible for biopsy. Cutting needle biopsy was performed in 124 cases, and fine needle aspiration biopsy was performed in 14 cases. In all cases, 14–22-gauge bio-psy needles were used (a mean of 20 G). The size of the punctured lesions varied from 11 to 115 mm.
A GE LightSpeed VCT, 64-row scanner (GE Healthcare) was used for all the examinations and biopsy planning. The examinations were performed using the helical scanning technique. The custom settings adapted to reduce the radiation dose were used. The tube voltage was 80 kVp; the mA was set to 30-150; the beam collimation was 40 mm; the slice thickness was 0.625 mm; the scan range covered the lung area 10 cm cranial and 10 cm caudal to the needle; the scan rotation time was 0.4 s; and the automatic exposure control (AEC) was activated. Adaptive Statistical Iterative Reconstruction (ASIR) was used (50% ASIR and 50% of FBP). Patients were positioned according to the location of the biopsied lesion (supine, prone, right/left lateral decubitus) and stabilized with straps. If the risk of complications was low and the patient could be positioned flexibly, the prone position was preferred. This respiratory movement of the chest wall prevents the patient from seeing the needle. Using a laser and coordinates from a low-dose CT scan, the skin and subcutaneous tissue above the biopsy track were anaesthetized with a 5-10 ml dose of 1% lidocaine administered with a 23-gauge needle. The biopsy was performed using the CT coordinates without the use of fluoroscopy to avoid radiation exposure of the personnel.
The lung lesion specimen was aspirated into the needle cavity and then spread evenly in a circle approximately 1-2 cm in diameter on a microscope slide for subsequent cytological analysis. In addition, a portion of the visible tissue fragment obtained from the glass slide or from the excision biopsy specimen was placed in a single container filled with formalin for subsequent histological exa-mination.
The qualification process for a patient’s biopsy involved a series of steps: initial thoracic surgical and radiological case review, obtaining patient consent, conducting a preoperative low-dose CT scan, performing the low-dose, CT guided biopsy procedure with rapid follow-up low-dose CT biopsied lobe, and concluding with a subsequent radiological follow-up. A chest X-ray was performed for uncomplicated biopsies and a low-dose lung CT scan for complications.
Results
The diagnosis confirmed by biopsy was obtained in 111/135 (82.2%) patients. In 97/135 (71.8%) cases, the histology results indicated the presence of neoplastic cells. The predominant histological types were adenocarcinoma 33/135 (24.4%), non-small-cell lung cancer 19/135 (14.0%), and unidentified atypical cells 26/135 (19.1%). No neoplastic lesions were found in 14/135 (12.6%). Nearly one in five patients 24/135 (17.7%) did not undergo a diagnostic biopsy and therefore underwent further diagnostic examinations. The results are presented in Table 1 and Figure 1.
Table 1
Diagnostic performance of low-dose, CT-guided biopsy
Complications were observed in 31 patients (22.9%), comprising 26 cases of a small pneumothorax requiring no further intervention and 2 cases of pneumothorax necessitating surgical intervention and drainage of the pleural cavity. Other adverse events included 3 cases of pleural or mediastinal haematomas that did not require medical intervention (Figure 2).
Figure 2
Computed tomography-guided thoracic biopsy of non-small cell carcinoma located in the right upper lobe. Axial (A) and sagittal (B) reconstruction
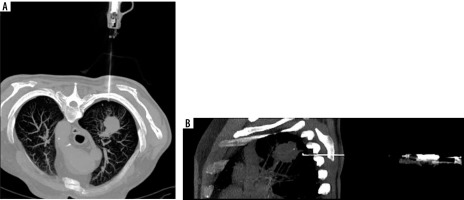
Figure 3
Number and types of complications that occurred during the procedures (pneumothorax was classified as clinically relevant based on the necessity of surgical intervention)
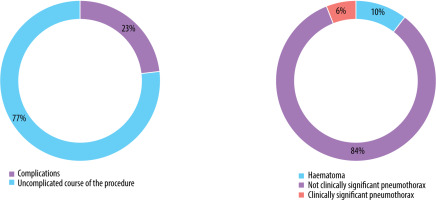
Figure 4
Complications occurring during the procedures. A) Minor bleeding in the biopsy canal. B) Not clinically significant pneumothorax
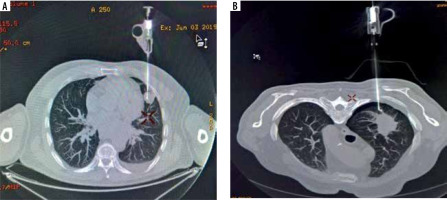
A low-dose protocol for CT-guided thoracic and pleural biopsies was used in this study. The mean DLP achieved during the procedure was 719.8 ± 441.1 mGy cm with a median of 586.0 mGy cm and the mean CDTIvol was 35.3 ± 18.1 mGy with a median of 35.3 mGy. This dose included a whole-lung scan before biopsy and a follow-up scan of the affected lobe after each biopsy.
Discussion
Low-dose CT transthoracic lung and pleural biopsy is considered as a safe, effective, and minimally invasive dia-gnostic modality for the evaluation of lung and pleural lesions [9]. The most common complication of this procedure is pneumothorax. The incidence of pneumothorax is estimated to be 25.3% of all procedures [11]. However, due to the self-limiting nature of this phenomenon, drainage is required in approximately 3.5-6% of cases [10]. Factors indicating a higher risk of pneumothorax occurrence include advanced age of the patient, smaller lesion size, no history of surgery, and penetration of more than one visceral surface of the pleura (e.g. when passing through the interlobular fissure). Some studies classify COPD and emphysema as such risk factors [12-14], although other research has found no significant association between these conditions [15]. Strategies commonly used by interventional radiologists to reduce the risk of pneumothorax include positioning the patient in the prone position with the puncture site down, using fluoroscopy, and administering a sedative [16,17]. However, some studies suggest that a prone patient position may lead to systemic air embolism [18]. The second most common complication is pulmonary haemorrhage, with an incidence of approximately 18% [4]. The established and most frequently reported risk factors contributing to the haemorrhage are as follows: (1) lesion related (e.g. size, location, histopathological diagnosis), (2) patient related (e.g. age, gender, morbidities), and (3) technology related (e.g. needle angle, duration of the procedure, number of needle adjustments). The incidence of the aforementioned complications affects the patient’s recovery time, prolongs the length of hospital stay, and is directly related to the cost of treatment. In addition to adjusting the patient’s position during the procedure, other attempts have been made to reduce the number of adverse events. Promising results in this area have been achieved with the use of needle puncture plugging introduced by McCartney et al. in 1974 [19]. Since then, various materials have since been tested for this purpose; namely, hydrogel plug, normal saline, fibrin glue, and haemocoagulase, with encouraging results in further minimizing potential complications [4,20,21]. Other potential complications occurring during the procedure include haemoptysis, needle tract seeding, systemic air embolism, haemothorax, and death [5,6].
The most common complication in this study was pneumothorax, which occurred in 28 (20.7%) of the patients. However, drainage was required in only 2 (2.2%) cases due to deterioration of respiratory parameters and clinical signs of dyspnoea. Haematomas were diagnosed in 3 patients, but these did not require further intervention. No other significant complications were reported during the procedure or at follow-up X-ray examination, demonstrating the safety of the procedure. The complications observed are consistent with the findings of pre-vious studies by Lim et al., who reported pneumothorax in 27.3% of patients, and Covey et al., who documented pneumothorax in 23.4% of participants. The incidence of pneumothorax requiring surgical intervention at our centre was similar to that reported by Lim et al., at 2.2% of cases, whereas Covey et al. reported a higher proportion of severe complications, at 6.8%. Differences in the prevalence of pneumothorax documented in the aforementioned studies may be due to a larger sample size.
The utilization of low-dose CT-guided biopsies has become routine clinical practice. A CT-guided percutaneous transthoracic core needle biopsy is typically performed at 100-120 kVp tube voltage. A low-dose CT protocol reduces radiation exposure to both the patient and the medical staff. In addition, the use of low-dose CT maintains satisfactory image quality, as shown by numerous studies reporting comparable levels of accuracy between low-dose CT protocols and standard protocols [22].Morosetti et al. reported a total of 45 ultra-low-dose CT-guided needle biopsies of pulmonary lesions (80 kV, 3 mAs). The DLP was 186.71 mGy cm. The overall technical success rate was 82.22%. The authors noted 2 major and 21 minor complications. Major complications required further treatment and hospitalization. The authors found a significant correlation between complications and node size. The authors state that this protocol is effective and safe for patients [10]. Frisch et al. reduced the tube voltage to 80 kV with a fixed exposure of 20 mAs and found that this gave similar results to the 100 kV tube voltage. A significant reduction in radiation exposure was also shown, measured at 34 mGy cm. As the authors point out, this difference represents a more than 10-fold reduction in radiation dose [22]. Kallianos et al. added that in their study, the mean biopsy dose decreased by 64.4% with the low-dose protocol, from a mean of 319.7 mGy cm to 113.8 mGy cm. The protocol consisted of 3 phases. For the target phase, the protocol included 2.5 mm slice thickness, 100 kV for patients weighing < 140 lbs with arms up and 120 kVp for patients weighing > 140 lbs with arms down. In terms of milliamperes, 20 mA was specified for patients weighing < 140 lbs and 25 mA for patients weighing > 140 lbs [8]. In our series, we reduced the tube voltage to 80 kV and 30-150 mAs. The mean DLP was 719.8 mGy cm, marginally exceeding the reference dose suggested for diagnostic CT of the chest (336-478 mGy cm), but lower than the diagnostic reference level (DRL) for coronary CT angiography (430-914 mGy cm) [23]. However, the mean DLP in this study includes both the preoperative low-dose CT scan for planning and the postoperative low-dose CT control scan (both approximately 150-200 mGy cm). This configuration gave image quality that is directly related to biopsy effectiveness, to be maintained at a level comparable to that seen in related studies. The 82.2% success rate of the procedure achieved in this study is consistent with the 82.2% reported by Morosetti et al., and slightly higher than the 76.5% reported by Kallianos et al. However, this marginal variance may be due to classification differences. Furthermore, the demonstrated performance underscores the need to improve material preservation techniques and to encourage greater collaboration with pathomorphologists in order to optimize the effectiveness of the procedure. Moreover, constant development and evolution of CT scanners and reconstruction techniques such as ASIR and Deep Learning Image Reconstruction (DLIR) should push the researchers to lower the radiation dose even further and improve the currently utilized protocols while maintaining high procedure effectiveness.
This study has several limitations. Firstly, the biopsies were performed by a single group of attending radiologists within one institution. In addition, there was a lack of a standardized dose group for meaningful comparison. Finally, the study was retrospective in design, which precluded the collection of prospective data and the implementation of randomization.
Conclusions
Low-dose, CT-guided transthoracic lung and pleural bio-psy is a safe and effective technique. The procedure is associated with a low risk of serious complications. The most common complication is pneumothorax, which is predominantly self-limiting and rarely requires additional medical intervention. Despite a lower dose, this protocol shows little to no difference in procedural efficacy (in terms of obtaining material suitable for histopathological examination) compared with the standard procedure.